Beyond the scientific method: Philosophy in the biology curriculum
- Christian Moore Anderson
- Oct 14, 2020
- 8 min read
Updated: Nov 30, 2024
During my teacher training we had to write a masters' level essay on the implementation of the How Science Works component of the English secondary science curriculum. There was a bit of confusion as to what this really was, and many schools seemed to view it as a bolt-on exercise to appear intermittently (if at all) to tick boxes. It was seen principally as the 'scientific method', which meant to most people 'how to design and carry out an experiment'.
Furthermore, there was conflict in the curriculum between getting through an overload of content and the idea of uncertainty in science. For example, How Science Works sought to instill an idea of the messiness of experiments, results, and analysis in science, but this ran against the conventional purpose of the science practical: for students to experience what the teacher wants them to experience, and hopefully to avoid it going wrong and wasting sacred curricular time. The How Science Works concept appeared to make for contradictory teaching; one minute a teacher requires the correct answer, and the next they want the student to know that there isn't a correct answer, just messy experimental results.
Back then I thought that was the extent to philosophy in the secondary science curriculum. I was wrong. The philosophy of biology goes far beyond experimental techniques, and well beyond the difference between correlation and causation. Rather than making intermittent appearances, it transcends the curriculum.
While biology is the study of life, the philosophy of biology is the study of biology itself. It was only when I began reading philosophical pieces did biology really begin to make sense to me. My curricular design, my vocabulary, my explanations and expectations, my vision changed, because it made me reflect on what I knew, and what that meant, and how that translated to my pedagogy. So how does the philosophy of biology appear in the curriculum?
In this post I give a very brief overview of some ideas that have made me reflect and change my practice, and I'll link to accessible sources for teachers. I will typically put the most accessible at the top of each list.
Beyond the scientific method
First of all, it's important to disentangle the idea of the scientific method and experimental methodology. Firstly, there is no scientific method. There are many methods used by scientists and the most important of which is purely descriptive work. Just as Alfred R. Wallace collected and described for years the animals he encountered and their distributions in the tropics. Typically, descriptive work provides the data with which scientists think, and infer. Darwin's and Wallace's natural selection was an inference. Experiments are just one tool (often employed to support a body of evidence for theories). Once an inference has generally been accepted as the best explanation of observed phenomena it is typically referred to as a theory.
Good reading:
Norman Lederman, 2018. Nature of scientific knowledge and scientific inquiry in biology teaching. In Teaching biology in schools, edited by K. Kampourakis.
Daniel Thurs, 2015. Myth 26: That the scientific method accurately reflects what scientists do. In Newton's Apple and Other Myths about Science, edited by Kampourakis.
The uncertainty of our models
Reality is too complex for a human to understand. Thus, biology is an endeavour to make sense of complexity in life, during which only the most important details are considered. A model is produced to attempt explanation, and if it is a good fit it is useful, but it will never be reality, because all the minor details were removed. This makes uncertainty inherent to all our models.
In biology education we teach models. Mendelian inheritance is a model, the digestive system is a model, evolution by natural selection is a model. The models of how replication and fertilisation occur are from model organisms (not humans). They are all simplifications that cannot capture reality, but do a decent job at approximating it for students.
I now begin my curricula with a discussion of the idea of limitations in science. Students need to know that they are learning models that offer explanation, they are not learning about raw reality. For example when teaching the particle model (if I may give a none-biology example), our first topic in Year 7, students often ask some questions that the model doesn't quite explain. Rather than stating to a student that they will learn about it later, it is a great opportunity to return to the philosophy of science.
As such, food chains will be presented, not as reality, but as highly simplified models, as are food webs (albeit more complex than a chain), and other biological models, such as r/K selection, levels of organisation, et cetera.
The image below is what I use in my first lesson with Year 7 (with some help from google maps). For those looking for a very accessible book to begin thinking about including these ideas in their curriculum, a good starting point is Uncertainty by Kostas Kampourakis & Kevin McCain.
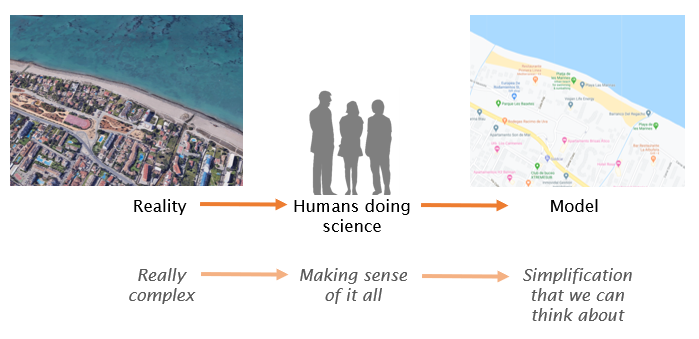
Of course, the google maps satellite image isn't reality either, but it approximates the truth enough for understanding, and hence, the image above is, in itself, also a model.
Good reading:
Explanation
As teachers, this question is of upmost importance. It does not pertain to pedagogy, such as how to explain in the classroom, but to the reflection upon what an explanation in biology really is. What is explanatory and what isn't. Are all explanations in biology about what causes what? Or can there be other explanations? Ernst Mayr made an early attempt in categorising two types of explanation in biology: ultimate and proximate. For Mayr, proximate questions were about how biological organisms function, how they carry out phenomena, and how they develop. Ultimately however, why they exist is a question of evolutionary history.
Nevertheless, this is continually debated by philosophers of biology, and ideas are always evolving. Despite categorising explanation into ultimate and proximate, there is still a lot of room to debate what those explanations would necessarily entail to be counted as explanations.
An important realisation for me was that explanations and models in science often contain idealisations that cannot be true, such as frictionless models in physics, ecological models that assume an infinite population size, and the ideal gas law. Thus, explanations in science will often sacrifice accuracy for the ability to generalise to many contexts (although the opposite can be true depending on the needs of the explainer).
This provides a deep insight: biology does not seek the truth, but to seeks to explain, and as such, my biology curriculum is not designed around what students need to know, but what they should be able to explain about life.
Of course, explanations are dependent on knowledge and the syllabus content of biological knowledge is unlikely to be affected. What this insight changes however, is how I organise that knowledge in my curriculum, and how I talk about that knowledge with students. Students should be aware of the scientific enterprise and the limitations of even our most favoured models.
For example, food webs are highly simplified models that show feeding relationships but omit many details such as the degree of feeding, and non-feeding relationships. Ecology is highly complex and although we can portray some of this by considering the effects of extinction within a community, if students are to understand the extent of the complexity we must discuss what is not shown in the model, and provide some concrete examples. Dead oak tress, so elegantly promoted in Isabela Tree's Wilding, as essential ecological elements for habitat creation, are a good example.
Another example would be the relationship between genotype and phenotype. Mendelian genetics is a model that is as simplified as possible in biology education to the extent of the inheritance of one gene and its associated trait. The model is useful in that is helps students understand how inheritance is particulate, but unhelpful in that the simplification cannot explain organismal phenotype. Therefore, before teaching the Mendelian model, I discuss with students the complexity in phenotype that we readily observe, even in eye colour, and how that translates to complexity in genetics. Then the model can be presented as a means of explaining inheritance patterns, rather than explaining observed phenotype.
Good reading:
Kostas Kamourakis & Kai Niebert, 2018. Explanation in Biology Education. In Teaching biology in schools, edited by K. Kampourakis.
Angela Potochnik, 2020. What constitutes an explanation in biology. In Philosophy of science for biologists, edited by K. Kampourakis.
Angela Potochnik 2017. Idealization and aims of science. (This one is deeper, if you like that).
Epistemology & ontology
Epistemology is the study of knowledge, in other words, how we can know. It concerns us here as it is important to reflect on how we make sense of biological phenomena as what we interpret to be knowledge will affect our curricular planning and explanations. For example, what is a gene and how should we define it at different stages of education? Often we use metaphors to help ourselves and other make sense of phenomena, but are they always appropriate? Talking of genes as 'blueprints', or Nature Vs Nurture, for example could lead to unsatisfactory beliefs about the nature of human society.
In my blog posts I talk a lot about levels of organisation, but do levels really exist? Can you really ascend the levels, or descend the levels, when really the molecular level exists everywhere? And, why is the molecular level at the bottom? Reflecting on these matters has allowed me to better explain these ideas in the classroom.
An ontological question will consider if something is actually a natural entity of the universe or not. And so, are levels of organisation ontological, or only epistemic (a way for us to organise our knowledge)? Are species real entities, and if so, what about sub-species? On reflection of rampant interdependence and symbioses, do individuals exist or are they just a manner for humans to make sense of life? What do we mean when we use the word function in biology if organisms have not been designed? Does it matter if many concepts in biology are developed in the realm of botany and zoology, and do not fit well in microbiology? What is life?
These are the questions are really enjoy, and they have changed the way I see biology and my biology curriculum. The latter point is important, how we reflect on our models, definitions, and metaphors will affect how our students' sense-making.
As teachers we have to decide how we organise our own knowledge, so that this can shine through in our curriculum to provide a 'way of seeing' for our students. A good example here is the ongoing debate between Richard Dawkins and Denis Noble, both of which have access to the same data, but interpret it in different ways. Dawkins ascribes to a view of DNA controlling a vehicle (the cell) to favour its own propagation, whereas Noble ascribes to a view of the cell using DNA as a resource.
Therefore curricular design should begin with what the overarching philosophy will be, and how that will be reflected in my lessons and discussions.
Good reading:
Kostas Kampourakis, 2020. Why does it matter that many of biological concepts are metaphors? In Philosophy of science for biologists, edited by K. Kampourakis.
Evelyn Fox Keller, 2010. The mirage of a space between nature and nurture.
Kostas Kampourakis, 2013. The philosophy of biology: A companion for educators.
Ayala and Arp, 2010. Contemporary debates in philosophy of biology.
My books: Difference Maker | Biology Made Real, or my other posts.
Download the first chapters of each book for free here.