How I teach the lac operon (without PowerPoint)
- Christian Moore Anderson
- Oct 12, 2024
- 5 min read
Updated: May 11
The lac operon is a classic of genetics and is common in biology curricula. Yet, students can sometimes find it difficult. I want to show you how I've taught it using diagrams and dialogue. You can read about the benefits of teaching without PowerPoint here.
I began by discussing the structure of the lac operon. This lesson came after several in which we'd considered the complexity of eukaryotic genomes. This included introns and vast regions of "non-coding" DNA. Yet bacteria have evolved to be streamlined. I drew the diagram below to show how the genes are positioned.

Bacteria have different pressures than eukaryotes. When their genomes become too large, their reproduction rate decreases as they take too long to replicate their DNA. Here we see three genes, shoulder to shoulder, under the regulation of a single promoter.
To help my students see the meaning of this I varied the concept. I contrasted it with a hypothetical genome in which each gene were isolated and controlled by its own promoter regions. The genome would be larger, and coordination would be tougher. Here, the genome is organised to efficiently synthesise three proteins as a package for a single function: obtaining and metabolising lactose.
Efficiency, then, is key for the bacteria, in more ways. I explained to my students that a bacterial population that continually produced these proteins, even when no lactose were present, would be wasting precious resources that could be used elsewhere. A system must regulate the gene expression so that it is expressed only when needed.
I introduced them to the repressor in the diagram above and then drew the next:
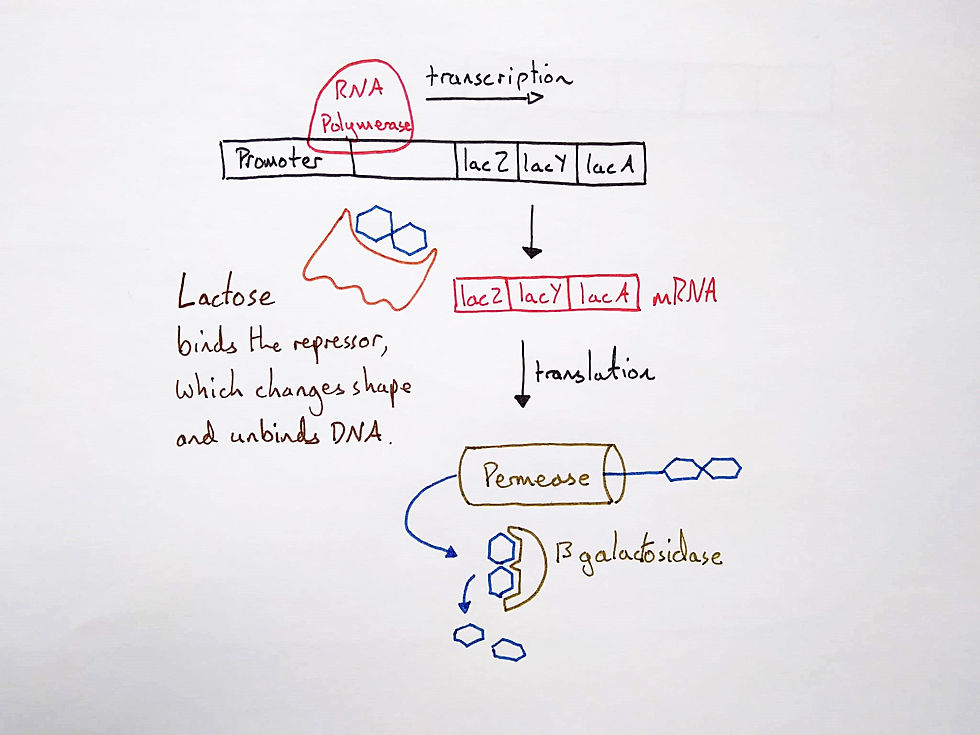
Now I explained the basics of the lac operon. Lactose binds to the repressor, which changes shape to lose its affinity to DNA. RNA polymerase is then free to transcribe a single mRNA yielding three proteins. I told the students we'd only focus on two: the membrane pump (permease) and the enzyme (beta-galactosidase).
With these two diagrams, the students could see the varying conditions of the system: repressed or activated. But, they also needed to consider the system dynamics over time. This is where I introduced a stock and flow model. I began by drawing the cell with the stocks and flows inside.

I then asked the students to name the flows, which they did relatively easily except for one. Interestingly, they wanted to name the outflow of the mRNA stock "translation". I asked the class to vote to see if they agreed, and they did. This gave me a key insight into how they were understanding the model.
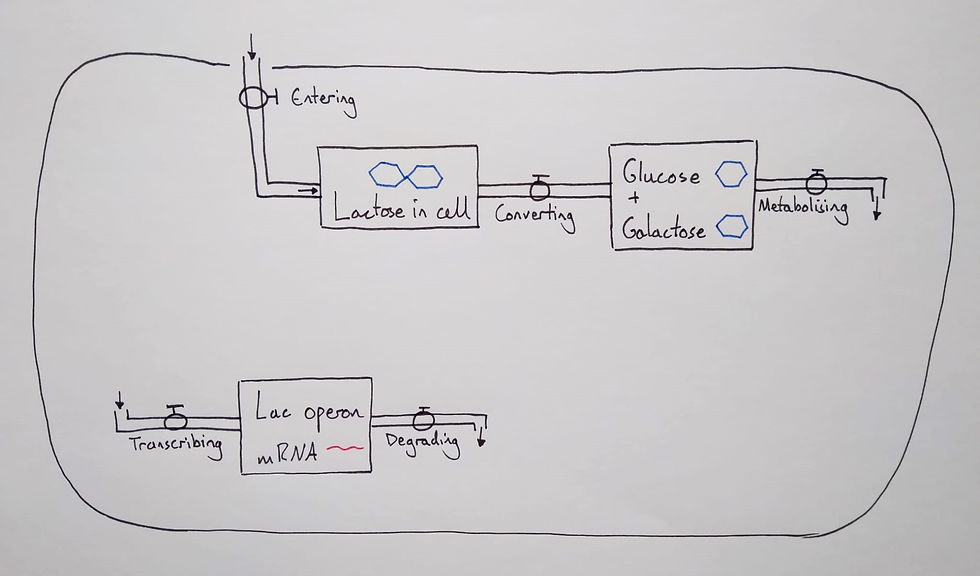
I mentioned that ribosomes produced proteins and asked if the ribosomes also destroyed the mRNA. After a brief discussion, they understood their mistake. I asked them again for a name of the outflow and we settled on "degrading". This was a good opportunity to discuss the nuclease enzymes that continually remove mRNAs from the transcriptome and the natural half-life of RNA.
For the next step, I asked the students what regulated the transcription of the operon. They answered that the repressor did by looking back at the diagrams of the operon. I added this variable to the diagram (below) and asked the students whether the connection was "same" or opposite".
Same "+": More X, more Y; or less X, less Y. Opposite "–": More X, less Y; or less X, more Y.
I gave them some time to think and then they voted. They agreed that it should be "opposite" (negative). In other words, the higher the repressor's affinity (for binding DNA) the lower the transcription rate.
And so what regulated the repressor? They agreed that it was the presence of lactose. I added the connection from the lactose stock to the repressor and asked them again to vote whether it was "same" or "opposite".
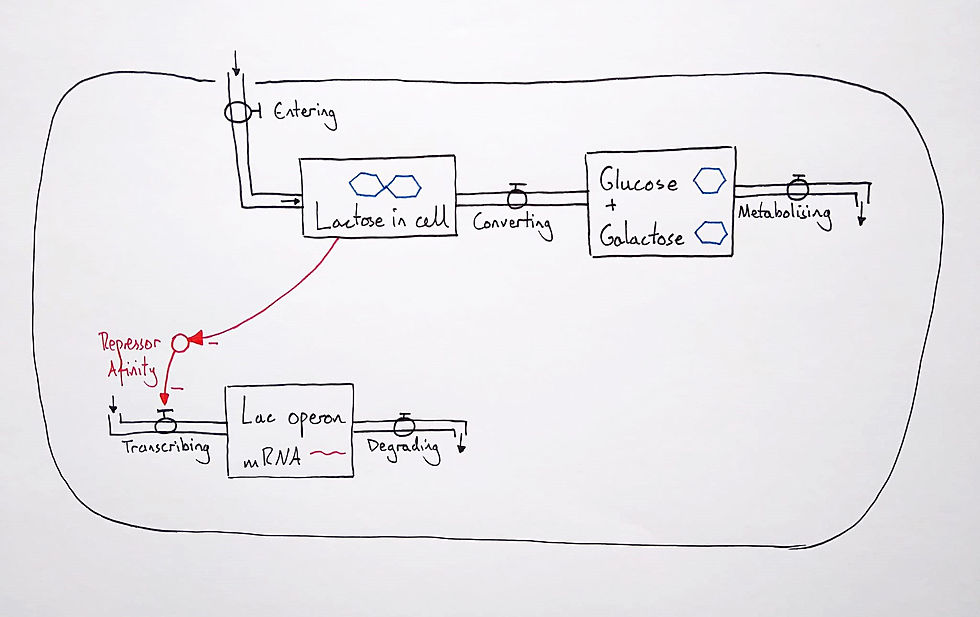
Now we had sufficient variables in the model to consider some dynamics. I told the students there was lactose in the cell. If we follow the connections, what would happen? I let the students think and asked for answers. Of course, the lactose would inhibit the repressor, transcription would proceed and there would be an accumulation of "lac operon mRNA". This would be transcribed into the proteins we'd already mentioned. I added these to the diagram:
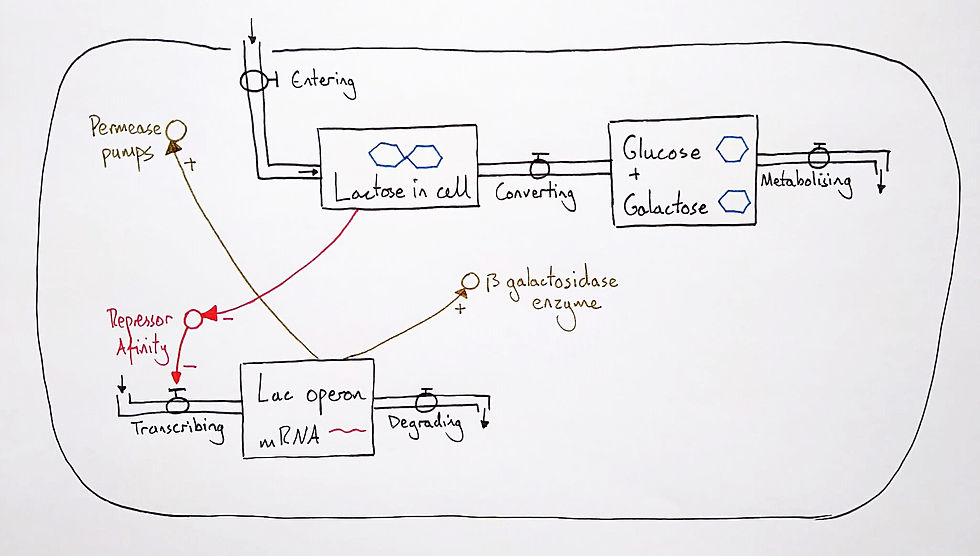
I focused next on the membrane pumps and added them to the diagram. I asked the students what the rate of "entering" would depend on. They suggested the number of pumps and the concentration of lactose molecules outside. Therefore, I added these two connections to the "entering" flow. Both have a "same" connection, because, for example, "the more lactose outside, the more will enter."
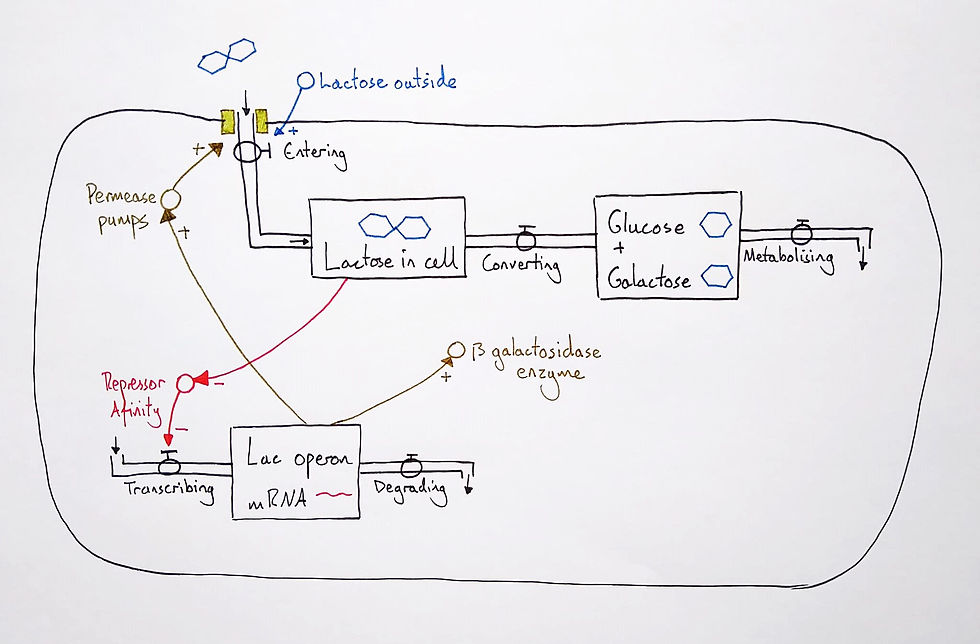
We then traced the lactose through the system (using fingertips always helps). Lactose would enter and accumulate in the cell. Then it would be converted into glucose and galactose. The focus now turned to the enzyme and we added the "same" connection to the "converting" flow.
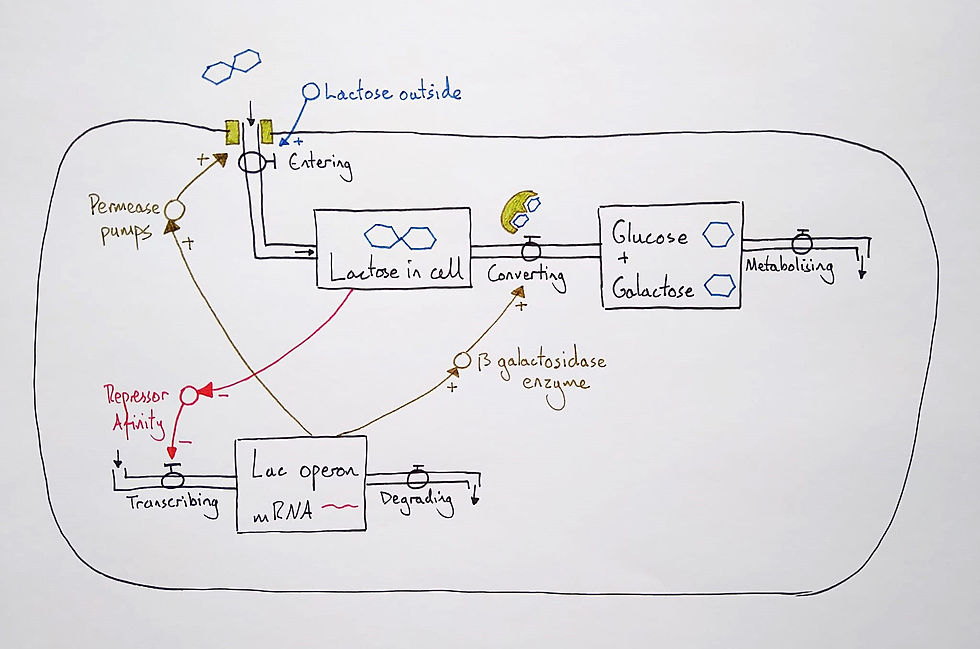
The model was now complete but there were still more dynamics to explore through variation. I asked the students what would happen if the lactose concentration outside started to diminish. Following the model over time, they agreed that the lactose inside the cell would decrease. This is because less would be coming into the cell than being converted into glucose and galactose.
This would then cause the repressor to free itself of lactose, regain its binding affinity for DNA and shut down the expression of the operon. The system seemed to work and the students appeared satisfied. But, I asked them, if a new source of lactose was encountered, what would happen?
The students fell for the trap. They began tracing the lactose through the system as if it could enter immediately. After some time, some students pulled faces as they realised something wasn't quite right. If the operon was not expressed, there wouldn't be any pumps in the membrane and, therefore, lactose couldn't enter to inhibit the repressor.
Once they had noticed the problem it was time to give my explanation. I pointed out the variable had been named "repressor affinity". I told them the affinity wasn't "100%" but a bit less. This means that, just by chance and probability, the repressor would unbind spontaneously from time to time. This means that a very low level of the operon mRNA is generally always present, and, therefore, so are the pumps often present in the membrane.
To give this system meaning, I varied the concept. I contrasted it to a hypothetical system that had to synthesise proteins that would detect the presence of lactose and then somehow communicate it to the genome. This would likely have a higher energy cost. Leaving it to chance, in this case, works well enough. We finished the lesson with the students self-explaining the model. This is teaching with diagrams and dialogue; do you want to co-construct meaning without lecturing, slide decks, or leaving students to discover for themselves? Learn how to teach the new way in Difference Maker.