During my training year as a teacher I taught one lesson that went really well with a bottom set science class. It generated a lot of engaging discussion for a typically quiet and disengaged class. Most of it was centred around the question of inheritance and whether specific characteristics, such as your height, presence of freckles, eye colour, et cetera, were the result of genetic or environmental inheritance. Now, I look back with regret.
It just seems so intuitive to everyone (probably the reason for my successful lesson); those in China learn to speak Chinese, but had they been born in Spain they'd speak Spanish. On the other hand I look quite like my dad, and in the end of the day we're all born looking human with four limbs. There does seem to be a divide between the effects of genes and the environment, but which is, of course, breached by the classroom examples of how both genetics and lifestyle causally affect your height or your skin colour.
But let's consider this point in more detail. There appears, intuitively, to be two entities that affect the phenotype of an organism. By bringing the idea to the classroom that these two entities, genetics and environment, impose their influence on us then we further instill a dichotomy. When we then consider examples of where both play a role to modify phenotypes the dichotomy is further entrenched. Hence the title of Evelyn Fox Keller's book (2010) The Mirage of a Space between Nature and Nurture.
The nature versus nurture debate is an old paradigm that is perpetuated in popular culture. These gaps between theoretical biology and society need to be addressed; the new models need to permeate the secondary classroom (Moore, 2013).
But this isn't just about changing perspectives of biological phenomena, ideas of inheritance inevitably spill out into society and the social world. And so, what happens when students are to receive the message that it could be genetics or environmental, or both. How does that idea apply to body composition, intelligence, or even race or gender? There is a danger here, just as with Mendelian genetics, that we can inadvertently ingrain (erroneous) genetic determinism: your genes are who you are.
So what are the new models and what changes could we see in the biology curriculum?
How phenotype arises
While several people have mentioned nature and nurture, the first person to demarcate the terms in an exclusive manner was Francis Galton, Charles Darwin's cousin, famous for his founding role in the ideas of eugenics (Keller, 2010). Galton set the tone for many years to come and shaped the debate that has continued through the 20th century.
The problem is that with our current understanding of gene expression the idea of genetics contributing something without the input of the environment just doesn't make sense. As Keller (2010) puts it:
'The casting of the debate as an effort to determine ''how much of our behaviour is driven by our genes versus the environments in which we grow up or live'' poses a question that is not only unanswerable but... actually meaningless.'
The reason it is meaningless is because genes cannot exist without an environment. Gene expression is triggered via cues from the environment (be that intra or extracellular). An organism simply cannot develop without the right information triggering the correct sequence of gene expression. A gene without an environment is just a molecule. It becomes a gene only in the right context:
'... a gene requires that the sequence be embedded in a cellular complex that not only reads, translates, and interprets that sequence, but also defines it, giving it its very meaning.'
Therefore, questions about how much of a characteristic is due to genetics cannot be made. The following analogy by Ned Hall shows us why (from Keller 2010, and annotation added in Moore & Shenk, 2016):
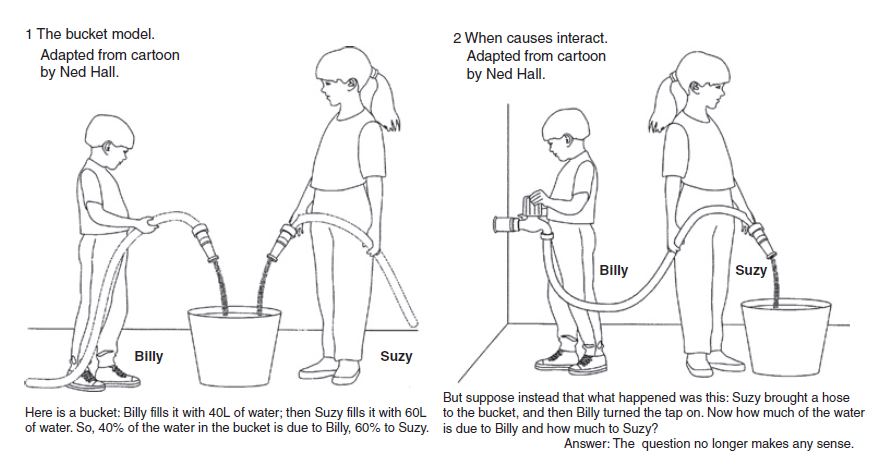
As such, information flows from the environment and the cellular equipment reacts to it to regulate gene expression. And despite the events such as the Human Genome Project, we still haven't learnt much about what genes do, because it is just so complicated.
We have our celebrated examples that appear in common biology curricula, such as mutations that result in sickle cell disease, phenylketonuria, or cystic fibrosis, but really these just tell us how an observed difference in phenotype correlates with a few specific mutations.
The presence or not, the difference between individuals, does not tell us anything about what causes the difference in phenotype. To understand the cause of the disease it is a biochemical analysis that is required, not necessarily a genetic analysis (Keller, 2010). And yet still, in most cases this tells us little of the gene's role in the 'normal' state.
There's more, these celebrated examples are exceptional; they are the simple ones. The relationship of gene products to phenotype is so convoluted that 'the effect of changing a variable that is itself known to be causally important to the production of the phenotypic end product may be (in fact, often is) reduced or erased by a system of buffering that is built into the dynamical networks mediating between genotype and phenotype.' (Keller, 2010).
In their paper titled The Heredity Fallacy, Moore & Shenk (2016) give a useful analogy to understand why differences in phenotype cannot be explained by differences in genes:
'One winter, in a particular neighborhood, there is a rash of house fires. Committed to fixing the problem, the city sets out to determine what caused the fires. They gather as much data as they can about all the homes in this fire ravaged neighborhood—including those that had fires and those that did not. They find that 100% of the fire variation in the group is attributable to whether or not space heaters were present in the various homes.'
But this difference does not explain the cause, because 'it turns out, every single home in this particular neighborhood was built out of highly combustible wood and painted with highly flammable paint.'
And just before I move on to consider what this means for the biology curriculum, I'd like to leave another gem from Keller's book (2010):
'DNA doesn't do anything. It does not make a trait; it does not even encode a program for development. Rather it is more accurate to think of DNA as a standing resource on which a cell can draw for survival and reproduction, a resource it can deploy in many ways, a resource so rich as to enable the cell to respond to its changing environment with immense subtlety and variety. As a resource, DNA is indispensable; it can even be said to be a primary resource. But a cell's DNA is always and necessarily embedded in an immensely complex and entangled system of interacting resources that are, collectively, what give rise to the development of traits.'
What does this mean for the biology curriculum?
Ultimately, Keller believes that the perpetuation of the idea of the dichotomy and its associated language is a result of the early classical gene definitions of a gene codes for a trait that continue to permeate society.
Burian & Kampourakis (2013) argue that the current definition of a gene has become so complicated and elusive to clarity that it is probably best to avoid it entirely and use the more inclusive term genetic material, which also avoids perpetuating the myth of a direct association between genes and phenotypes. They say that:
'One could then base the discussion on the evolution of genetic material, the interaction between the genetic material and its intracellular or extracellular context, and the expression of genetic material to produce RNA, proteins or other molecules...'
But more than just a change of language I think there should be a change in curricular structure. Currently I perceive it to be common to have whole topics in lower-secondary biology named Variation. In which we often find a lesson, at least, on inheritance and the contribution to phenotype by genetics and/or the environment.
Maybe it is time for the focus to shift. Rather than contributions to variation, the perspective could change to developmental plasticity and how the flow of information between the environment and the genetic material can result in a multitude of varying phenotypes.
It is to change the question itself so that it shifts away from causality, away from the false dichotomy, and towards:
Which traits are more or less malleable than others? and,
Up to what point in development do particular characteristics remain plastic? (Keller 2010).
There are a number of advantages with this new emphasis beyond the limitations of what science can and can't answer, and these include the avoidance of instilling genetic determinism.
Including phenotypic plasticity in the curriculum
Having some simple examples of how the plasticity of organisms may improve not only student understanding of phenotype but also of evolution (Kampourakis & Stasinakis, 2018). It also moves the focus of biological reasoning away from a gene-centric narrative, and to something more dynamic and complex.
Kampourakis & Stasinakis (2018) argue that if students can see how change can occur during the life of an organism (developmental time), they may be more likely to appreciate how change can occur over generations (evolutionary time) in population lineages.
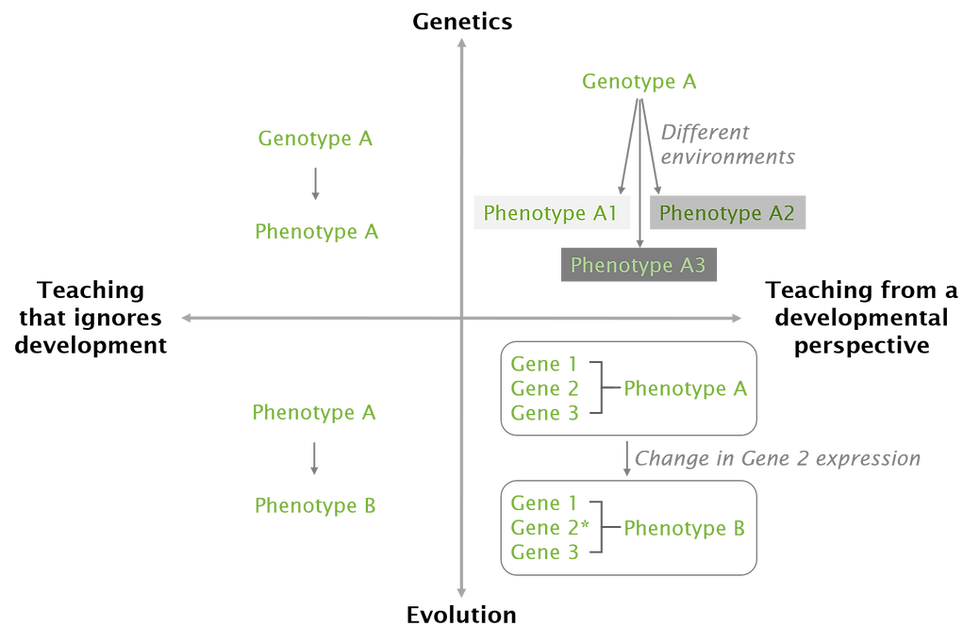
Image adapted from Kampourakis & Stasinakis (2018)
Kampourakis & Staskinakis (2018) recommend that students don't have to learn about developmental biology per se, but a few concrete examples to show and promote an understanding of (amongst others):
Development (the growth and transformations that occur during life cycles)
Developmental robustness (the ability to develop into the adult form of a species irrespective of the environment, such as having four limbs in humans)
Developmental plasticity (the ability of organisms to show phenotypic variation depending on the local environment)
They suggest that the first two points should be introduced earlier than developmental plasticity. This makes sense and is in concordance with the resources of Best Evidence Science Teaching, that firstly the concept of species should be explored first—lest students get confused about the sameness of morphology observed in monophyletic lineages.
Let me just finish with productive examples that can be used in lower secondary:
One of the easiest examples that can serve as a discussion point is to grow plant clones in differing conditions, such as soil quality or lighting conditions. This can serve as a practical of a simple thought experiment and act as a spring board to discussing animals. For animal behaviour, one of the most famous examples that never fails to create interest is the effect of the quantity of grooming that a mouse pup receives and the consequences on the pup's behaviour as an adult. Those who receive more attention show more tendency to be calm and less nervous, and also to nurture their own pups in a similar fashion.
However, some of the most fruitful discussions I've had have been in upper secondary when we've discussed Sex Chromosome Aneuploidies. Simply asking students to predict the phenotype of, say, an individual with XYY, or XXY, reveals many students who see organisms as genetically determined. Many times they predict that the individuals will produce both reproductive systems side by side. Another productive example I've discussed with students is an individual who is XY but whose testicles do not descend and become deformed inside the abdomen, such that their primary sexual characteristics to not develop in a typical way.
I'm still looking for good examples to discuss with students, so if you know of any. Get in touch. Let's see if, as Keller hopes, the nature vs nurture question can just fade away.
My books: Difference Maker | Biology Made Real, or my other posts.
Download the first chapters of each book for free here.
Christian Moore-Anderson
@CMooreAnderson (twitter)
References
Burian, R., Kampourakis, K., 2013. Against “Genes For”: Could an Inclusive Concept of Genetic Material Effectively Replace Gene Concepts? In K. Kampourakis, ed. The philosophy of biology. London: Springer, pp.597-628.
Kampourakis, K., Stasinakis, P, K., 2018. Development. In K. Kampourakis & M. Reiss, ed. Teaching biology in schools: Global research, issues, and trends. London: Routledge, pp.99-110.
Keller, E.F., 2010. The mirage of a space between nature and nurture. Durham: Duke University Press.
Moore, D., 2013. Current thinking about nature and nurture. In K. Kampourakis, ed. The philosophy of biology. London: Springer, pp.629-652.
Moore, D., Shenk, D., 2016. The heritability fallacy. Wiley interdisciplinary reviews. Cognitive science.