What are enzymes? For students that's a strange word. So, firstly, we'd better have a look. To do so, my favourite animation is one of lactase by biointeractive. Not only is the animation nice, but it's a context that is familiar to students. Here's how did it recently with students 14–16 using diagrams and dialogue, culminating in a stock and flow model.
Key distinctions
Yet, a distinction must be drawn before we can explore that context. After watching the animation, we watched it a second time and discussed what's going on. I told them that enzymes are proteins, and then I asked a question: What is bigger, a cell or an enzyme? I had them vote by raising their hands. As anticipated, many thought enzymes were larger.
This was a common confusion; some students took a while to discern between the different "invisible entities of the body". To help them, I returned to the organic molecules. I drew a cell, as shown below, and asked students what they were made of. They replied that they were made of organic molecules.
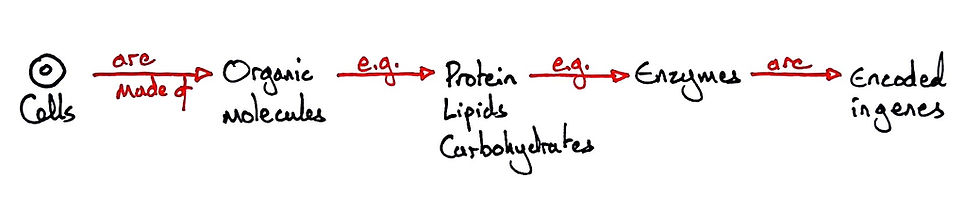
I asked students for examples of those organic molecules and wrote some down. I then reminded them that enzymes were a type of protein encoded in genes.
"So, which is bigger, cells or enzymes?" I asked again. This time, they voted for cells. But we weren't finished yet. I asked, "How many enzymes do you think fit into a typical cell?" The students offered small numbers, like 100, or 1000, maybe 1,000,000. I told them there could be billions. This gave them a better sense of scale.
There was another key distinction that students typically confuse. This time I asked: "Are enzymes alive?" And some students thought they might be. After watching the animation, I suppose, they may have perceived causative action and related it to life. I then returned to the idea of cells being the smallest living unit. With this rule, enzymes can't be alive, they're just molecules.
Putting Enzymes in Context
We were ready to return to the context that gives purpose to the concept of enzymes. The reason this context is nice is because it involves a natural contrast—a difference, variation—that students are familiar with. As a class, we'd discussed lactase's function, but what if it didn't function?
I taught the students about lactose tolerance, why it likely evolved (see this video) in two human populations and why the rest of the mammals are lactose intolerant after weaning. "What if you were lactose intolerant and drank milk, what would happen?" I asked.
The key is that the enzyme isn't present, so lactose isn't broken down, and therefore it can't be absorbed by the cells of the intestine. And if it isn't absorbed, it stays in the lumen and the bacteria there have a feast. Ultimately, the person would experience gases and diarrhea, (depending on the quantity of lactose).
"What about lactose-free milk... how could we make that?" I listened to some of the students' ideas, often more complicated than the answer, and then I told them. You get the milk, and you add lactase to it. So the only difference is that one contains lactose, and the other contains glucose and galactose (plus, maybe, some lactase).
The Mechanism of Enzymes
Now, my students were ready to tackle the mechanism of enzymatic reactions. I drew a diagram in three stages with my students, which I discuss next.
1. Enzyme and substrate
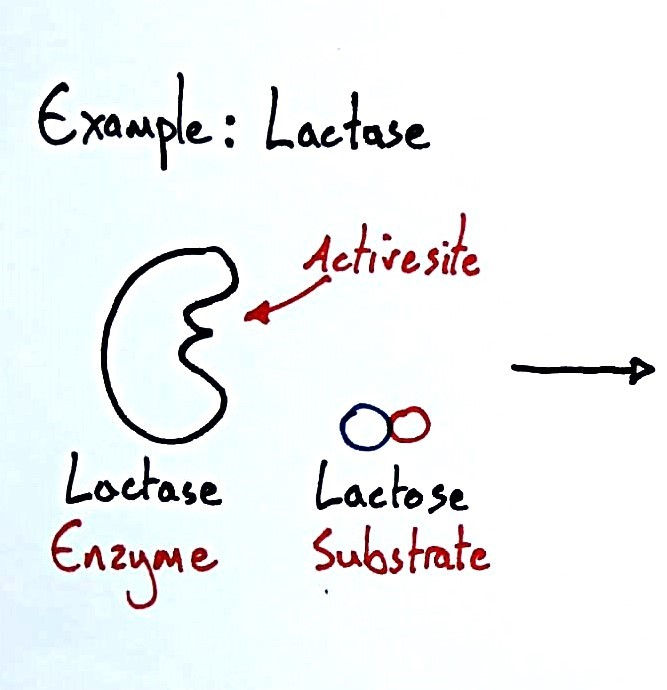
I drew the first diagram while the students drew with me. I then distinguished the example from the concept by writing "lactase" and "lactose" and telling the students that they were the "examples". We needed a name for the concept: lactase is a type of enzyme but what is lactose? An example of a substrate. I told the students that the part of the enzyme that was active—the part that carried out the reaction—was called the "active site".
2. Enzyme-substrate complex
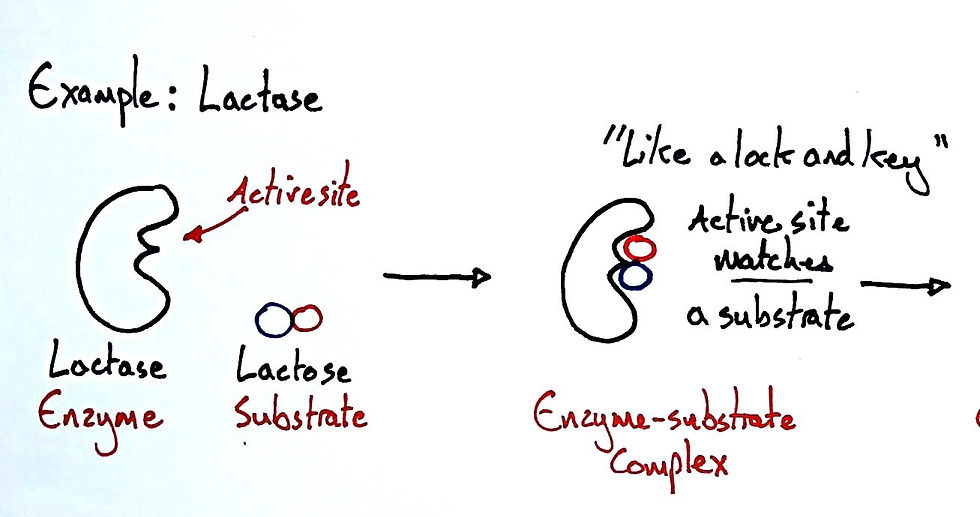
As they'd seen in the animation, I drew the substrate binding to the enzyme and named it. I then discussed the lock and key analogy. (I noted a crucial difference: locks don't tend to modify keys).
3. Enzyme and product

Finally, I drew the third step of the diagram. As with the first step, I discussed glucose and galactose as examples of "products". Enzymes don't always break down single substrates into different products, they can do the opposite. I gave the students an example: glycogen synthase (shown below).
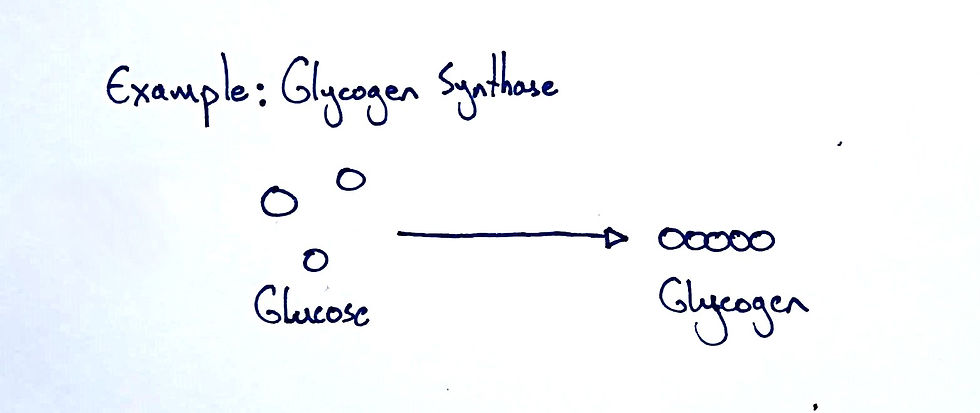
A Stock and Flow Model
I wanted the students to think deeper about what happens during enzyme-catalysed reactions and relate this to their central importance to the life (the autopoiesis) of cells.
To do this, I drew the model below and asked students to draw their own. I asked them to name the stocks and flows using the information in the diagram of the enzymes we'd already drawn (above).
After hearing some of my students' answers, I filled in my model and asked them to add in the answers (below).
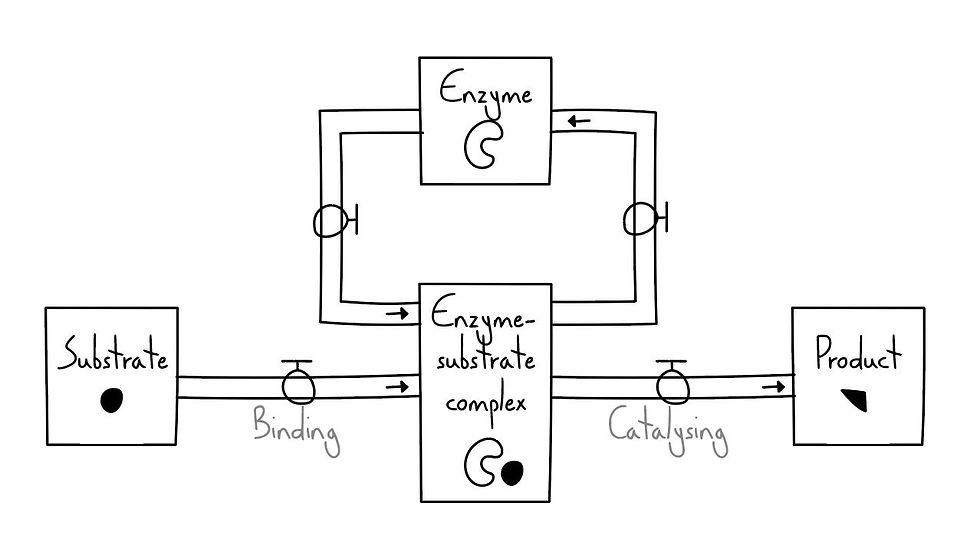
Key to this model is a fundamental distinction: the substrate changes (into the product) but the enzyme stays the same. I wanted the students to perceive this.
As always with these models, I asked students to trace the ideas with their fingertips. Firstly, I asked them to trace the substrate through the reaction. "What happens to the substrate?" It's converted into products. Then I asked them to trace the enzyme through the reaction in the model. "What happens to the enzyme?" It stays the same and is available for another reaction. A single enzyme can catalyse thousands, millions, of reactions
So what? What's the relevance? I then covered the enzyme stock with my hand so that students could only see the three stocks of the model.
I asked, "What if enzymes were used up in the reaction, what if each enzyme could only catalyse a single reaction?" "How many lactase enzymes would you need in the intestine after a glass of milk if each one was used up in each reaction?"
This contrast was related to our previous work on organic molecules and the relative expense of proteins due to their nitrogen content. "If every reaction were like this, how much protein would we need to consume to produce so many enzymes every minute? It would be impossible."
I then taught them the term "biological catalyst" and explained why it was central to life and autopoiesis. And, therefore, why enzymes are encoded in genes: so the memory—of how to construct these essential parts of the system—is retained through time.
This concluded my fifty-minute lesson. In the next, I taught my students about enzyme kinetics, e.g. the effect of temperature on their function. This is teaching with diagrams and dialogue; do you want to co-construct meaning without lecturing, slide decks, or leaving students to discover for themselves? Learn how and why in my books. Download the first chapters of each book for free here.