How typical biology curricula get it wrong: The need to contextualise components
- Christian Moore Anderson
- Feb 3, 2020
- 11 min read
Updated: Aug 11, 2024
Should mitosis be taught alongside meiosis or separately? Should organelles be taught together or separately across the curriculum? How about organ systems, is it better to have them side-by-side to compare like for like at the same scale, or would it be better to get one system dive deep into its levels? For the first biology topic of secondary school, which of these two brief overviews of knowledge sequences do you like the best?

For me the choice is clear, but let me explain why.
Levels of organisation in biological systems
The levels of organisation in biological systems span the molecular to the ecosystem, but let's focus on human biology in the secondary school setting. A useful scheme for this stage of education, which is sharable with students, is that organisms can be considered to be organised into the:
Organismal level (the macro level, visible to the eye, hence systems, organs, tissues)
Cellular level (the micro level, visible via light microscopes, hence cells and organelles)
Molecular level (the level of inorganic and organic molecules, hence DNA, CO2, etc)
However, most biology curricula/exam specifications isolate levels of a system; processes and mechanisms occurring at one level are considered in isolation to what is happening at other levels.
For example, cell biology topics in secondary school tend to present students with an array of specialised cells to learn (cellular level only). Maybe there will be a lesson on the cell organelles and their functions (cellular level only). In another lesson students may learn about the different organ systems of the human body (isolating the organismal level). In other topics we may find the molecular details of genetics isolated from the phenotype (organismal level), or the mechanism of mitosis isolated from higher levels, etc.
Nevertheless, sometimes a curriculum will stipulate the teaching of a specific example through which students can see how the levels of the system are indeed connected. Currently sickle cell disease is a popular example, or indeed cystic fibrosis. While they are good examples, I propose that systems, along with evolution, form the two sides of the coin of biology in secondary education and therefore they must be an intrinsic component of all biology topics.
Living organisms are best described as complex systems of interacting components that are organised hierarchically, and that evolve over time.
These are the big explanatory questions in biology, the why of evolution, and the how of the system. We need to train our students to think more about biological systems as without it, they cannot make satisfactory systemic explanations in biology and while they may be more pragmatic at times, they may only know to ask what for?
Think about protein shakes that intended for body builders. What are they for? For building muscle. But how do they work? Even on a simple level, to answer this we must be able to trace amino acids through several organs of the body. We must also have an understanding of the dynamic nature of muscle which is continually being broken down and rebuilt. We must understand how an amino acid will be incorporated into a protein, which will form part of the structure of the muscle cells, and that the muscle cells form part of the muscle. The protein, at the molecular level, has causal activity that affects the muscle at the macro level.
However, the currently common biology curricula (in the UK context at least) isolate ideas about systems, and they isolate their levels of organisation. I will argue here that by changing to a biology curriculum centred around systems and mechanistic explanations students will:
Learn biological components and their functions more easily.
Learn more explanations than descriptions (and therefore a correct epistemic view of biology as a science of explanation).
Be able to generalise biological knowledge more easily between differing contexts (such as between animals, plants, unicellular organisms, and ecosystems)
Be able to access more easily the abstract concepts in upper secondary biology curricula (such as how genotype relates to phenotype)
Biological systems-thinking: vertically and horizontally
Biological systems can be thought of as being composed of components (C) and their mechanisms (M) they perform. The interaction of these lead to resulting phenomena (P). Here's an example I've come up with:

Image by Christian Moore Anderson
Hmelo-Silva et al. (2017) created this 'CMP' framework to help students learn about systems and assess their work. Do students talk more about components, mechanisms, or phenomena? Additionally, do they connect components and mechanisms more on the same level, or vertically to higher or lower levels (micro and macro)?
In general students talk mainly about components in a descriptive manner, and the connections they make tend to be on a single level, unless explicitly taught otherwise (Hmelo-Silva et al., 2017). But what about connections between components?
Verhoeff et al. (2008) suggest that students find 'horizontal coherence' (connecting components of the same level) to be easier than 'vertical coherence'
Importantly, students are much more comfortable at the concrete organismal level. As students move away from this level, the level of abstraction increases.
On the systems side of the coin, explanation involves mechanistic explanations, and that means students must have the knowledge to link components, and mechanisms both horizontally, and vertically. They must be able to descend the levels of organisation to the molecular level and ascend once more to see the result on the system at higher levels.
They must have an idea of how an input to the system is connected to its outputs. How does the input of organic molecules and oxygen, result in the outputs of carbon dioxide, water, and the release of energy? For a proper mechanistic explanation, a student must be able to trace these molecules through the system, organs, cells, and back up again.
Systems-thinking has the power to unite understanding across the many biological fields, rather than being seen as a discipline of many different fields each with their own vocabulary and components. The concepts of biological systems are generalisable to all contexts. All life is organised into interacting molecules that form cells, and multicellular organisms share the organisation of tissues, organs, systems. They all the share the necessity of inputs from the environment, and the result of outputs.
Without the ability to conceptually think about organisms as dynamic systems, students will fall short of many threshold concepts in biology. Let me give some examples.
Students will probably not be able to trace the path of molecules through systems (think the carbon cycle, or metabolites in the human body), nor will they be able to satisfactorily explain homeostatic feedback mechanisms, such as flexible responses to exercise in human biology as these occur across levels of organisation.
They will also struggle to understand how it is the behaviour of proteins that form the cell, or how mitosis occurs due to a self organising system (without intentions or teleology), or how the flow of information is both, from the DNA to the cell, and from the environment to the cell. Indeed, in systems biology nothing makes sense except in the light of its environment, but to understand how environment shapes a biological system, a conceptual threshold must be passed (Meyer & Land, 2006).
Just as with evolution, systems biology is a concept that develops in the students' mind with time, with many examples, much discussion and exposure. While there are some topics in biology curricula that do quite well with systems biology (such as KS3 topics covering the cardiovascular, and respiratory systems in the context of exercise physiology), systems-thinking requires a presence in most topics.
The pedagogy of biological systems thinking
One of the most salient examples of systems-thinking in secondary school biology is relationship between the molecular genotype and the organismal phenotype. In fact, if students do not pass this threshold, they're likely to revert to the erroneous and deterministic 'a gene codes for a trait' (van Mil et al., 2016). Such thinking jumps straight from the molecular level up to the organismal level without anything in between.
Phenotype is the consequence of incredibly complex interactions between proteins that are translated from DNA, but that also regulate the DNA via information that comes from the the cells interactions with its environment. A single protein may have the simple role of placing a hydroxyl group on a single molecule. Others may have an important role of regulating many other genes. Whichever it is, a gene, or protein, cannot alone code for a trait. The interactions of proteins form a cell, but the interactions of cells form a tissue, etc, producing phenotypes at every level. If students have had little education in systems-thinking leading up to upper-secondary they will struggle to visualise these interactions and will probably just take the message that DNA codes for organismal level phenotype, and this leads to a dangerous belief in genetic determinism: 'You are what your DNA codes for'.
Knippels (2002) suggests that when tackling genotype-phenotype then it is more productive to use what she calls 'Yo-yo teaching' which is the continual yo-yoing between the levels of organisation to explicitly show how interactions cause changes at other levels. Specifically however, she advocates starting any yo-yoing at the organismal level, as this is the most concrete to students.
This is similar to Maton's Legitimation Code Theory. In his paper 'Making semantic waves' (2013) he suggests that learning benefits from oscillating between the concrete, and the abstract.
Both of which also relate to Ausubel's meaningful learning; new content must relate to some prior knowledge, otherwise it is rote learning. Just as Meyer and Land (2006) suggest that unless a threshold of conceptual understanding is crossed, students resort to a kind of mimicry.
Components only hold meaning in the context of the their interactions with other components within a system.
I think that such semantic waves, and yo-yo teaching should not be the preserve of genotype and phenotype relationships, but an important method for teaching systems-thinking generally in secondary biology. Indeed, this is often how biology research proceeds: A phenomenon is discovered and the mechanism is sought. Through methodological reductionism, the system is decomposed to its components and their mechanisms until finally being recomposed to see how it all fits together to produce the overall phenomenon (Bechtel, 2013).
I suggest that topics should revolve around providing students the opportunity to make mechanistic explanations. At the very least, biological levels of organisation should not be isolated to any one level. They need to be contextualised to something more concrete, which means that they should be situated within higher levels of organisation that make sense to students. The picture below shows how a sequence of lessons can isolate (A), or integrate (B) levels of organisation.
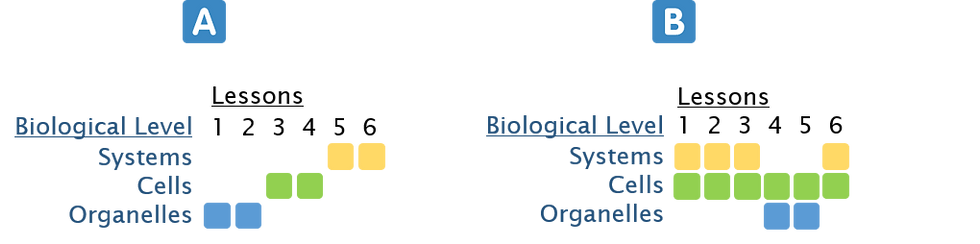
Image by Christian Moore Anderson
When those cell biology topics (at various stages) describe the organelles in animal cells and plant cells, not only are the levels isolated, but often they are described in generic cells, robbing them of any possible context. There is too much focus on description; biological organisms are composed of x components that have y functions. Students will learn more easily if components and functions are fully contextualised within a system's mechanisms.
Rearranging the content in topics for a systemic approach
Most of the commercial KS3 schemes of work I have seen, those found on TES, shared freely on the internet, or indeed the literal order found in the UK's National Curriculum (possibly the cause or the former examples), begin Year 7 biology with a (mainly) descriptive topic: Life is cellular, it has these organelles in these generic cells. Multicellular organisms have specialised cells and here are some examples with their adaptations. They are also organised into cells, tissues, organs, and then systems. Maybe also some example organ systems and their functions. Then popular knowledge-building activities may be used to instill this erroneous epistemic view of biology. In this manner knowledge can only be organised in disconnected and descriptive chains:

Alternatively, these contents could be arranged in a systematic, fashion that allows these components and their functions to be linked to the overall system horizontally and vertically. Thus the components are situated within an interacting network that allows students to connect them within a schema of knowledge for a specific mechanistic explanation.
For example, specialised cells in multicellular organisms could be taught alongside the organ systems they are found in and how the mechanisms of the cells affect the organ system (the higher level). Organelles can be taught inside specific specialised cells that students already know and within systemic mechanisms that contextualise them.
How the first Year 7 biology topic, Cells to Systems, can be rearranged
I begin with the requirements of cells in terms of exchange with the environment. What do unicellular organisms exchange in terms of matter? Dynamism and the flow of energy and matter are important aspects of biological systems and so I begin here, at the interface between the system and the environment.
Next step is to consider that life can also be multicellular and this is a good chance to consider some evolutionary why questions. But then we can step into consider why multicellular organisms are organised hierarchically, why do they need a circulatory system, etc? Now we can move into systematic how questions after a good discussion of diffusion constraints.
We can now consider a muscle cell (which is relatively concrete to a Year 7 student) and its requirements of exchange with the environment, and now we can move down to consider a new organelle, the mitochondria and its function. I've now set up the rest of the topic; a study of how the components on various levels of the system interact to ensure all cells have access to oxygen, organic molecules, and are able to rid themselves of carbon dioxide considering diffusion constraints. Finally the cell membrane can be brought in as the discussion arises about diffusion from plasma to body cells, or from the intestinal lumen and into the enterocytes.
The respiratory system and some of their specific specialised cells can be taught together, giving context to their function, as can the digestive system, and the circulatory system, etc. This means for example, that ciliated cells are taught when learning about the respiratory system and how their function works within the larger system. Here's a image to help convey my ideas:

Eventually students should have the possibility of attempting a mechanistic explanation rather than a descriptive account of human biology. They should be able to trace some molecules through the body passing through different components. They should have started on a good footing to begin seeing organisms as biological systems.
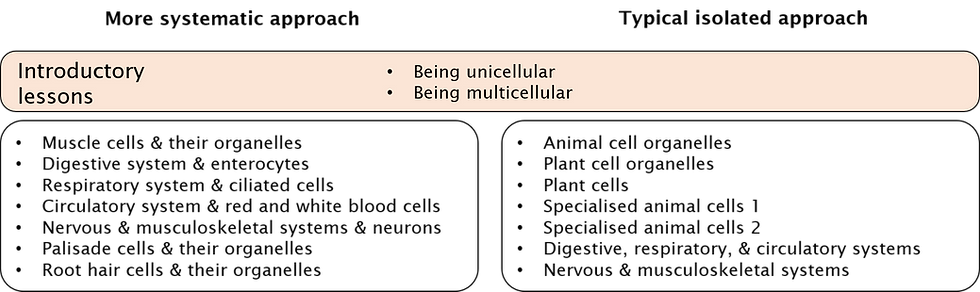
While systems in physics can be described minimalist, biological systems contrast as maximalist (Keller, 2010). They are incredibly complex, but that should not stop students from understanding the bigger concepts.
Systems biology in secondary education is not a reductionist endeavour, it is not about learning the minutia of a system. It is about allowing students to generalise conceptual ideas to all organisms, and living systems, based on knowledge of specific exemplar systems.
With consistent exposure and generous discussion of (and practice of) the big ideas in biology students should improve their conceptual understanding over time.
One question to ask when looking at biology topics is whether they allow students to form explanations, either evolutionary or systemic/mechanistic, rather than just a decriptive account of components. And, if there is a focus on systems, at what point the bigger conceptual ideas of systems will be discussed (see Ben Zvi Assaraf & Snapir, 2018):
Dynamism (the flow of energy and matter through the system)
Organisation (the hierarchically of organised levels)
Homeostasis (the maintenance of a constant internal environment via feedback)
There is little consensus (but several suggestions) to what systems-thinking is in biology education. However, in my pragmatic opinion, students should initially be making mechanistic explanations that include more than one level and tracing molecules through systems and across levels.
Next I would expect an understanding of feedback loops involved in homeostasis. Finally, students should be able to suggest how systems respond, on varying levels, to changing inputs (like intense exercise, for example). This last point for me is an important indicator of reaching a threshold in conceptual thinking. Students should be able to think of a system as dynamic and not static and thus be able to suggest, possible consequences of changes at one level on the other the levels.
My books: Difference Maker | Biology Made Real, or my other posts.
Download the first chapters of each book for free here.
Christian Moore-Anderson
@CMooreAnderson (twitter)
References
Bechtel, W., 2013. Understanding biological mechanisms: Using illustrations from circadian rhythm research. In K. Kampourakis, ed. The philosophy of biology. London: Springer, pp.487-510.
Ben Zvi Assaraf, O., Snapir, Z., 2018. Human Biology. In K. Kampourakis & M. Reiss, ed. Teaching biology in schools: Global research, issues, and trends. Londen: Routledge, pp.62-73.
Keller, E., 2010. It Is Possible to Reduce Biological Explanations to Explanations in chemistry and/or Physics. In F. Ayala, R. Arp, ed. Contemporary Debates in Philosophy of Biology. UK: Blackwell Publishing. pp.19-31.
Knippels, M.C., 2002. Coping with the Abstract and Complex Nature of Genetics in Biology Education: The Yo-Yo Learning and Teaching Strategy. Ph.D. Thesis, Utrecht University, Utrecht.
Hmelo-Silver, C., Jordan, R., Eberbach, C., Sinha, S., 2017. Systems learning with a conceptual representation: a quasi-experimental study. Instructional Science, 45, pp.53–72.
Maton, K., 2013. Making semantic waves: A key to cumulative knowledge-building. Linguistics & Education, 24(1), pp.8–23.
Meyer, J., Land, R., 2006. Overcoming barriers to student understanding: threshold concepts and troublesome knowledge, Abingdon: Routledge.
van Mil, M., Postma, P., Boerwinkel, D., Klaassen, K., Waarlo, A., 2016. Molecular Mechanistic Reasoning: Toward Bridging the Gap Between the Molecular and Cellular Levels in Life Science Education. Science Education, 100(3), pp.517–585.
Verhoeff, R., Waarlo, A., Boersma, K., 2008. Systems Modelling and the Development of Coherent Understanding of Cell Biology. International Journal of Science Education, 30(4), pp.543–569.