How I teach oxygen dissociation curves with a stock and flow model
- Christian Moore Anderson
- Dec 5, 2024
- 6 min read
Updated: Dec 7, 2024
Oxygen dissociation curves are difficult for students. I often find that difficulty comes from trying to visualise the mechanism through a graph. In this post, I'll show you how I've built a model of the system with a class, which we then translated into a graph.
Firstly, I began with two stocks and their flows. I labelled the stocks, situated them in a capillary (note the two red lines) and asked the students what the flows represented. They agreed that the first flows represented diffusion. The next two are deducible from the names of the stocks: binding and unbinding (I forgot to write the names on this diagram).
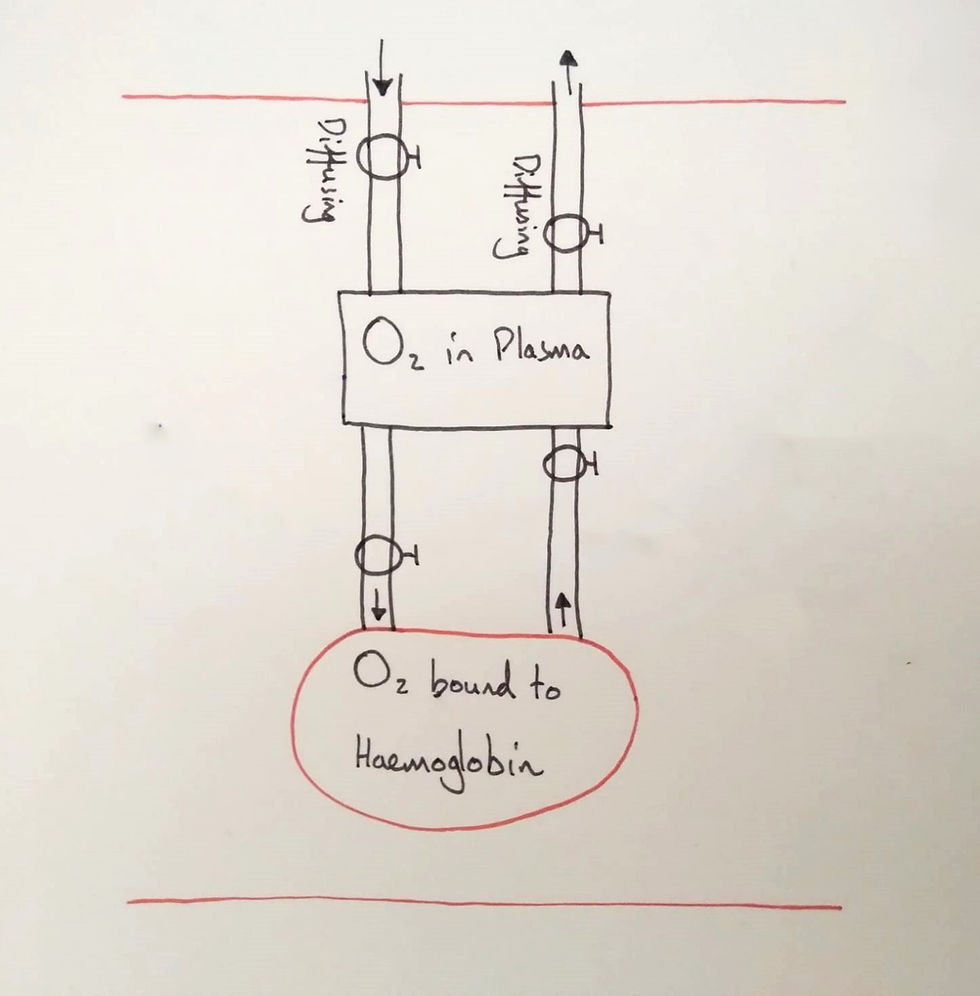
Fundamental distinctions
We'd already studied the alveolus and gas exchange so I told students that we wouldn't focus on the diffusion flows. Why would we focus on the other flows? To help students see this, I provoked them with a difference (a variation). I covered the haemoglobin stock with my hand leaving only the plasma stock visible and asked students why we had haemoglobin. This brought the role of haemoglobin into their awareness.
I told them plasma dissolved oxygen extremely poorly and is a very limited store. If we had no haemoglobin, then the heart would have to pump over 500 litres of blood per minute to deliver enough oxygen (compared to around just 5 litres). Haemoglobin, therefore, evolved to act as a large store.
I varied the idea. If we exercise, our bodies adapt to the demands by accumulating more haemoglobin in the blood. And, if we compare terrestrial mammals with diving mammals (e.g. whales and seals), the latter have a much larger quantity of haemoglobin compared to their body mass.
The difference between the oxygen content of the plasma and that bound to haemoglobin is a crucial distinction, but its implications need to be made clear. Any measure of the pO2 of blood is a measure of the plasma pO2.
This is also the case for chemoreceptors of the body, which uses the pO2 as a proxy for the oxygen content of the blood. But this can fail. To see it, I had to vary one of them. If carbon monoxide binds to haemoglobin instead of oxygen, the pO2 of the plasma should remain normal while the total oxygen stored in haemoglobin will be lower than normal (vastly lower in CO poisoning).
What interests us here, then, is how haemoglobin can accumulate and release oxygen within the body. I asked the students to discuss in pairs what affected the rates of the binding and unbinding flows.
One student mentioned temperature and another mentioned the concentration of oxygen. I told them that we'd group these two terms into one: pressure, and explained the term "partial pressure".

I added the variable to the model and the two connections and asked students if the connections were "same" or opposite".
Same: More X, more Y; or less X, less Y.
Opposite: More X, less Y; or less X, more Y.
The class agreed that the connections should be "same": the higher the partial pressure of oxygen, the higher the binding rate. I asked them why they thought that and the class discussed the probability of collisions between oxygen and haemoglobin.
I gestured two molecules with my fists, one representing haemoglobin and the other oxygen.
"So, you're saying that the more likely these collide, the more likely they'll bind." I then hit one fist into the other. "Now they've collided, what determines the binding now they are in contact?"
This produced a class discussion that led to the term "affinity" as a probability of bond forming and breaking. I added the variable to the model with the two connections and again asked the students to vote on whether they were "same" or "opposite".
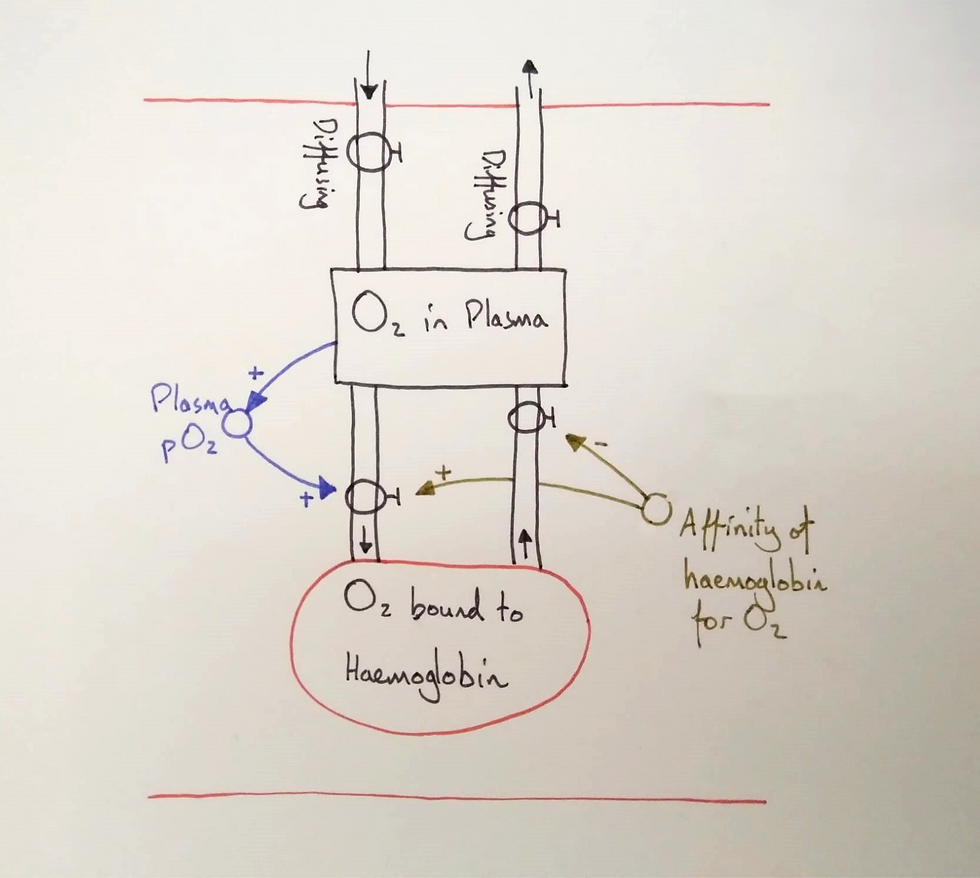
I asked the students if they thought the affinity for oxygen should be high or low and let them discuss it in pairs. This led us to agree that the affinity needed to be intermediate. High enough to bind oxygen in the pulmonary capillaries but low enough to allow unbinding in the systemic capillaries. This allowed us to vary the aspect of affinity and bring it into focus.
Cooperative binding
I told the students that affinity was added to the model because it can vary. I then explained how cooperative binding functioned (this image is useful). These extra details, such as conformational changes to haemoglobin, would make the model too complex to follow and so are taught separately to it. However, we summarised it as the affinity increased as more oxygen bound and we added it to the model.
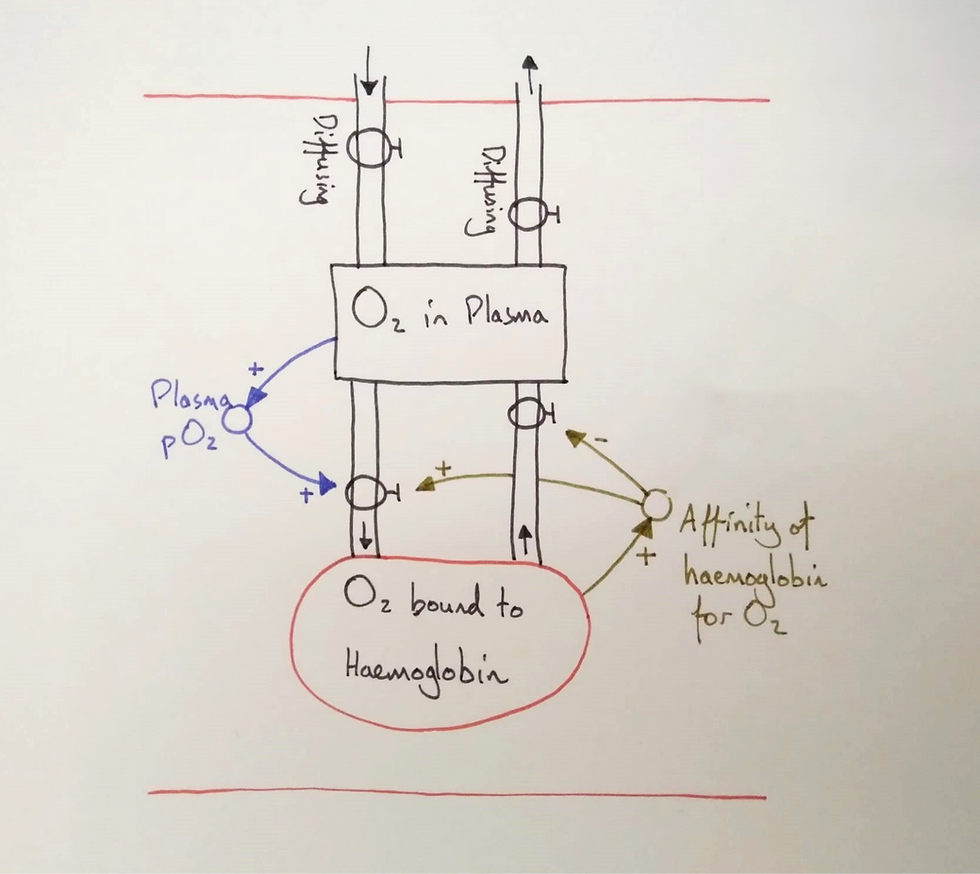
At this point, they hadn't fully made meaning of the role of cooperative binding. They needed to follow oxygen through the model and see how it translated into an oxygen dissociation curve on a graph.
Oxygen dissociation curve
We put our fingers on the model and traced the oxygen. Oxygen would diffuse into the plasma in the pulmonary capillaries but time is short. We traced the oxygen into the haemoglobin stock and noted the affinity increase. Thanks to this cooperative binding, the first oxygen molecules to bind open the molecule allowing more oxygen to bind more readily.
We then did the reverse for a tissue capillary of the systemic circuit. With less oxygen in systemic tissues, oxygen would diffuse out of the plasma stock. The lowered pO2 in the plasma reduces the probability of binding and as the first oxygen molecules unbind the affinity is lowered. This leads to a higher probability of unbinding and we traced the oxygen molecules to the plasma and then again as it diffused out.
I then drew the typical oxygen dissociation curve on a graph and discussed its shape.
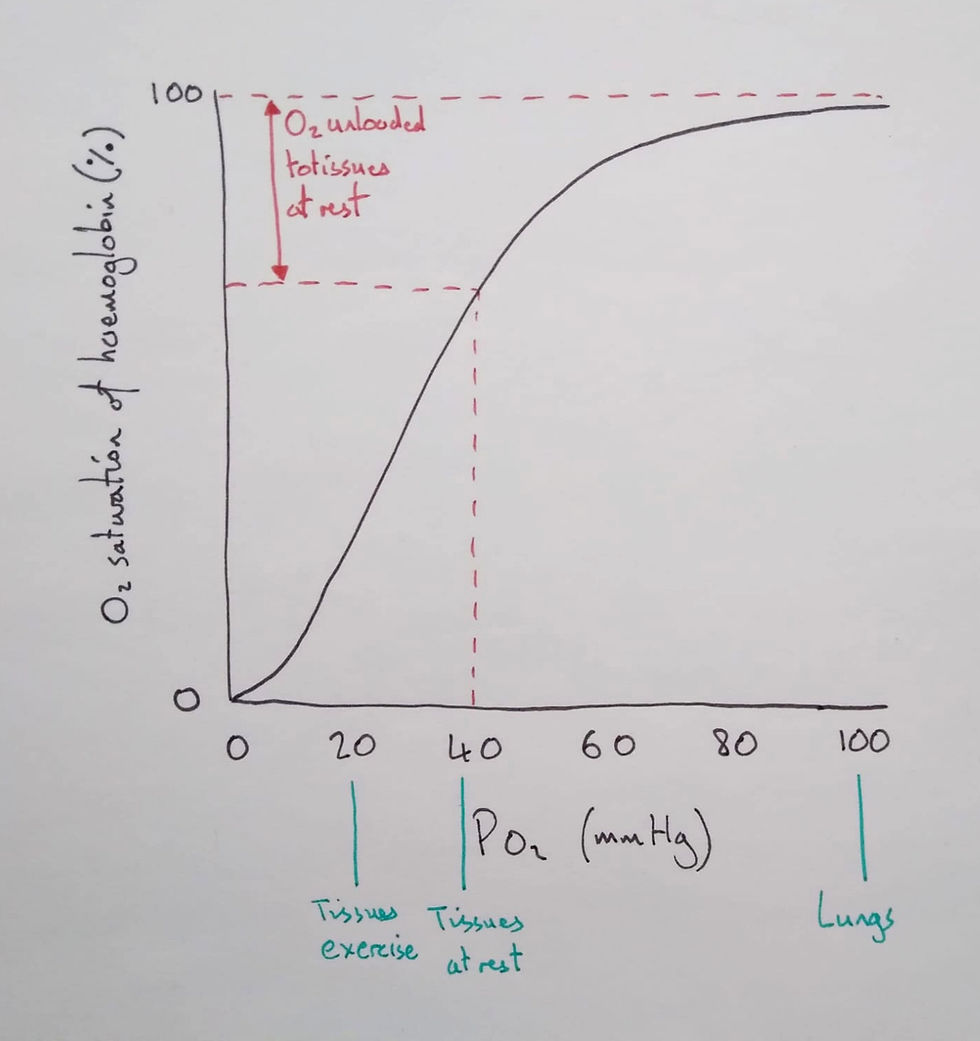
Students should distinguish between oxygen saturation (%) and concentration (ml of O2/100 ml of blood). I told them the percentage saturation is an average saturation of 1 to 4 oxygen molecules per haemoglobin. To know how much oxygen there is in total, you'd also need to know how many haemoglobin molecules there were in the sample.
To help students discern this, I varied one of the two aspects. If we compared an (otherwise healthy) anaemic person (less haemoglobin concentration) to a healthy person, they'd both have the same saturation at rest but the person with anaemia will transport less oxygen in total.
I then showed the students how to use the graph to see how much oxygen would be unloaded in tissues at rest and those engaged in exercise. We noted the shape of the graph and how the steep portion was due to cooperative binding. To finish this lesson, I let the students self-explain the diagram as if they were explaining to someone else.
Bohr Shift
I told the students that another variable affected the affinity of haemoglobin for oxygen, which helped it unload extra oxygen in tissues in demand of oxygen.
I explained how carbon dioxide reacted with water to form carbonic acid. Therefore, where carbon dioxide levels were high, the pH of the blood would decrease. The H+ ions would bind to haemoglobin, cause a conformational change, and lower its affinity for oxygen.
I added these variables to the model and asked the students to decide whether the connections were "same" or "opposite".
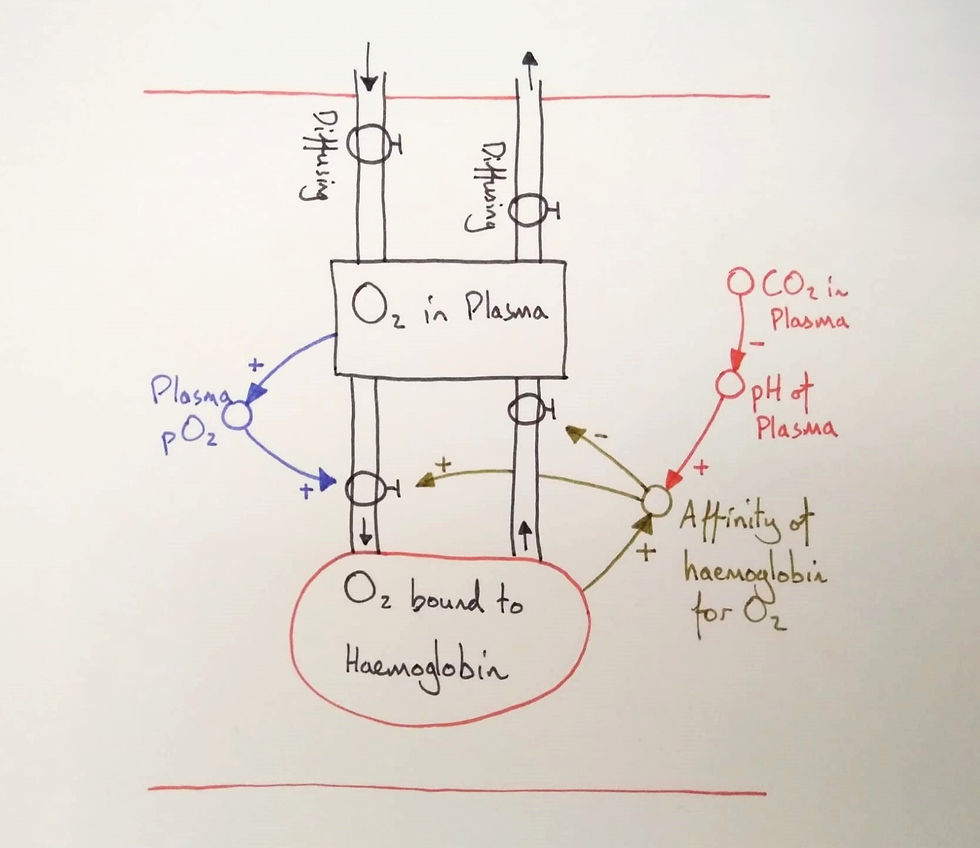
I probed the students: When the blood enters a systemic capillary, would the pH be higher, the same, or lower than in a pulmonary capillary? I had them vote by raising their hands. They agreed it would be lower but I asked why.
Through conversing we established that respiring tissues, which required oxygen, produced carbon dioxide. Therefore, a proxy for the oxygen demand is the quantity of carbon dioxide produced, or, the plasma pH.
We traced oxygen through the model again as the haemoglobin reached a systemic capillary. We put our finger on "CO2 in plasma" and began. A higher CO2 concentration leads to a lower pH, which causes a drop in affinity for oxygen and a higher probability that oxygen will unbind. It was time to see it on the graph.

For the same pO2, the Bohr shift shows that more oxygen is unloaded in tissues with a lower pH.
Finally, we discussed fetal haemoglobin and I added the line to the graph.
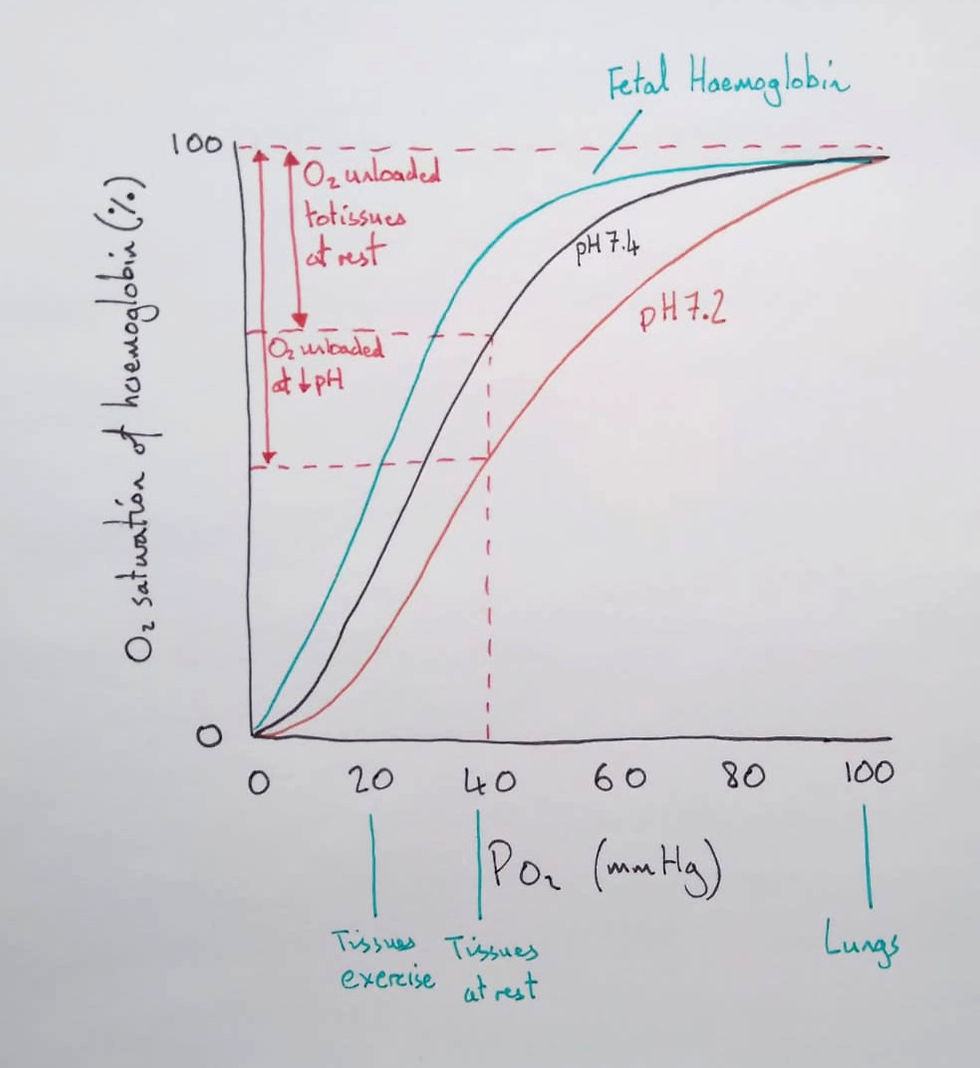
Yet, to understand this line, we returned to the model. We traced oxygen diffusing out of the plasma as it reached placental capillaries. The pO2 in placental capillaries is lower than in alveoli, and once oxygen has diffused into the fetal plasma, what's to stop it from diffusing back again? The fetus needs a mechanism that increases the probability that oxygen will arrive and stay in the fetal blood. For this reason, fetal haemoglobin evolved to maintain a higher affinity for oxygen.
Scroll down to see the final lesson I co-constructed with my students. Learn more about teaching through diagrams and dialogue – without lecturing, slide decks, or leaving students to discover for themselves – in my books. Download the first chapters of each book here.
